第一列
Among all the existing technologies to implement quantum computers, quantum photonic computers (QPC) are the only chip-scale solution to work at room temperature. Based on our recent demonstration on CNOT gate operation of photonic qubits with Si/Si3N4 waveguide circuits, in this project entitled “Integrated Photonic Quantum Computing Based on Non-Gaussian Continuous-Variable (CV) States with Error Code Correction”, we further combine the expertise of PI and co-PIs in integrated quantum light sources, quantum optics and metrology, programmable silicon photonic circuits, quantum state validation, quantum error code correction and error mitigation, and photon number resolving detectors, to form strong teamwork on the development of chip-based non-Gaussian CV state sources and the technology of Gottesman–Kitaev–Preskill (GKP)-state generation and metrology, serving as a critical step toward the realization of a large-scale fault-tolerant QPC.
This project proposes leading QPC schemes using advanced integrated photonic-chip technology in heterogeneous optical circuit platforms, which can be of great value to not only the scientific study of this exciting research field but also the technological development of practical quantum computers especially in Taiwan with well-established semiconductor industry.
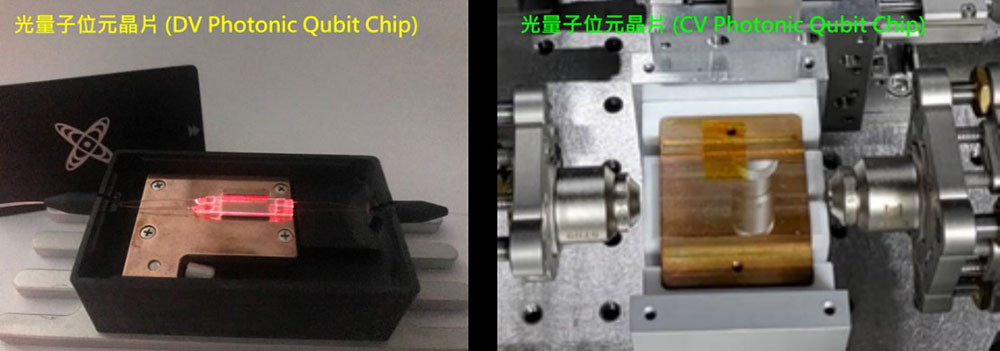
Photonic qubit chips developed by the research team of the project entitled “Integrated Photonic Quantum Computing Based on Non-Gaussian Continuous-Variable States with Error Code Correction”
-
Co-PI
-
Ray-Kuang Lee (National Tsing-Hua University)
-
Jun-Yi Wu (Tamkang University)
-
Shin-Tza Wu (National Chung Cheng University)
-
Yi-Shan Lee (National Tsing-Hua University)
Generation of heralded optical cat states by photon-addition
Optical cat states, the non-classical superposition of two quasi-classical coherent states, serve as a basis for gedanken experiments testing quantum physics on mesoscopic scales and are increasingly recognized as a resource for quantum information processing. Here, we report the first experimental realization of optical ‘cat states’ by adding a photon to a squeezed vacuum state, so far only photon-subtraction protocols have been realized. Photon-addition gives us the advantage of using heralded signal photons as experimental triggers, and we can generate ‘cat states’ at rates exceeding 2.3 × 105 counts per second. Our experimental implementation with controlled photon-addition demonstrates a new powerful building block for advanced quantum state synthesis.
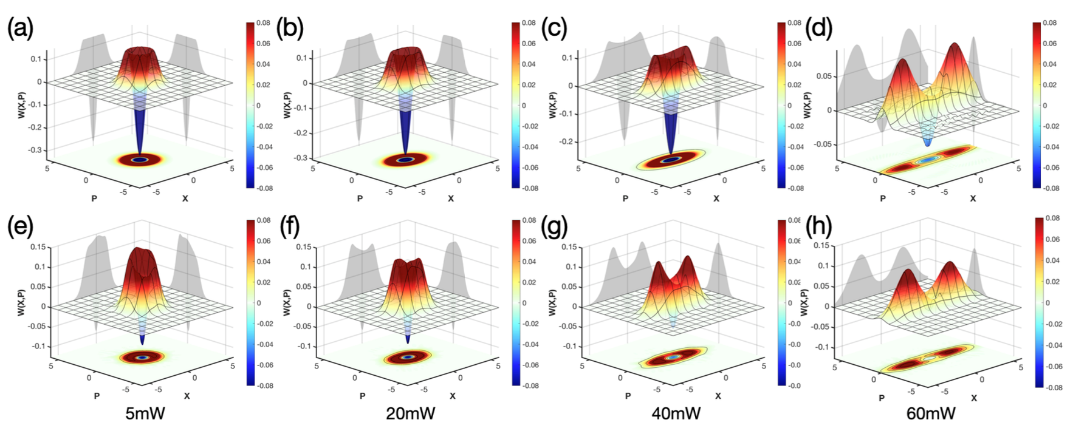
Wigner distributions of optical cat states: (a)-(d) for the theoretical photon-added squeezed states; and (e)-(h) for experimental data with 92% detection efficiency correction, obtained with OPO pump-powers at 5, 20, 40, and 60 mW, respectively.
Reference: Yi-Ru Chen, Hsien-Yi Hsieh, Jingyu Ning, Hsun-Chung Wu, Hua Li Chen, Zi-Hao Shi, Popo Yang, Ole Steuernagel, Chien-Ming Wu, and RKL, "Generation of heralded optical `Schroedinger cat' states by photon-addition," [arXiv: 2306.13011].
Time-Frequency Entanglement Distribution via Fiber Networks
Time-frequency entanglement has been highly regarded in quantum technology due to its high-dimensional entanglement and resistance to practical disturbances. In this work, we utilize a Franson interferometer to verify the time-frequency entanglement of photon pairs generated from spontaneous parametric down-conversion. We observed a high visibility of 94% in the nonlocal two-photon interference. We further demonstrate the advantages of time-frequency entanglement by distributing the two photons through a 2.64 km fiber network at the NCU campus, and achieve an interference visibility of 88.9%, which surpasses the classical limit of 70.7% by a significant margin.
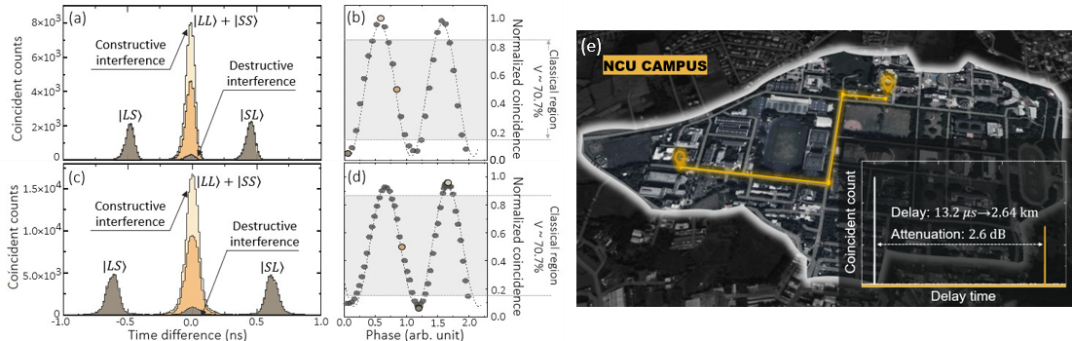
Two-photon interference in the unbalanced Michelson interferometer to demonstrate fiber-based entanglement distribution over a distance of (a), (b) 10 m in a laboratory and (c), (d) 2.64 km through the (e) NCU campus fiber network.
Reference: Optical Engineering, Taiwan Photonics Society, 158, 27 (2023)
Semiconductor Single-Pixel Photon Number Resolving Detectors (PNRD) Capable of Resolving Up to Five Photon States with a Figure of Merit of 0.99
In non-Gaussian quantum computing, a higher number of photons can be used to generate more complex and higher-intensity non-Gaussian quantum states, such as photon-subtracted states, photon-added states, or even cat states. Increasing the number of photons can significantly enhance the capability, stability, and precision of quantum computing, but it also introduces more technical challenges and equipment demands. For instance, it requires a single-photon detector capable of accurately resolving photon numbers while handling a high photon number state. Through device structure and circuit optimization, our work has significantly improved single-photon detection efficiency. Consequently, it has increased the photon number resolving capacity of traditional semiconductor single-pixel avalanche photodiodes from three to five photons, simultaneously enhancing the figure of merit for photon number resolution.
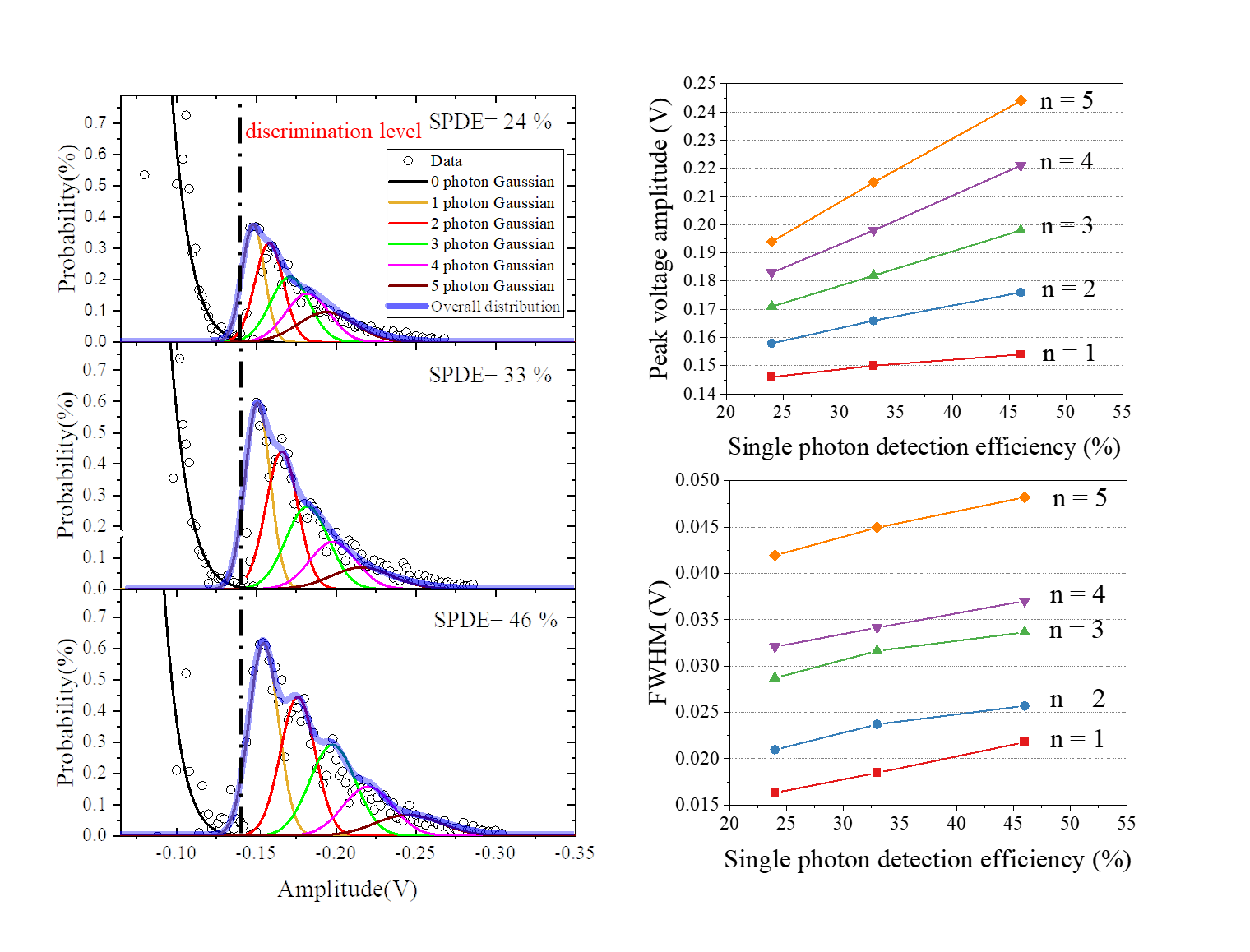
(Left) Distribution of the peak output signal at different SPDE values of 24%, 33%, and 46% at a temperature of 200 K. Each colored line shows the Gaussian fit to different photon number states.
(Right top) Peak voltage amplitude as a function of SPDE for different photon number states.
(Right bottom) Full width at half maximum as a function of SPDE for different photon number states.